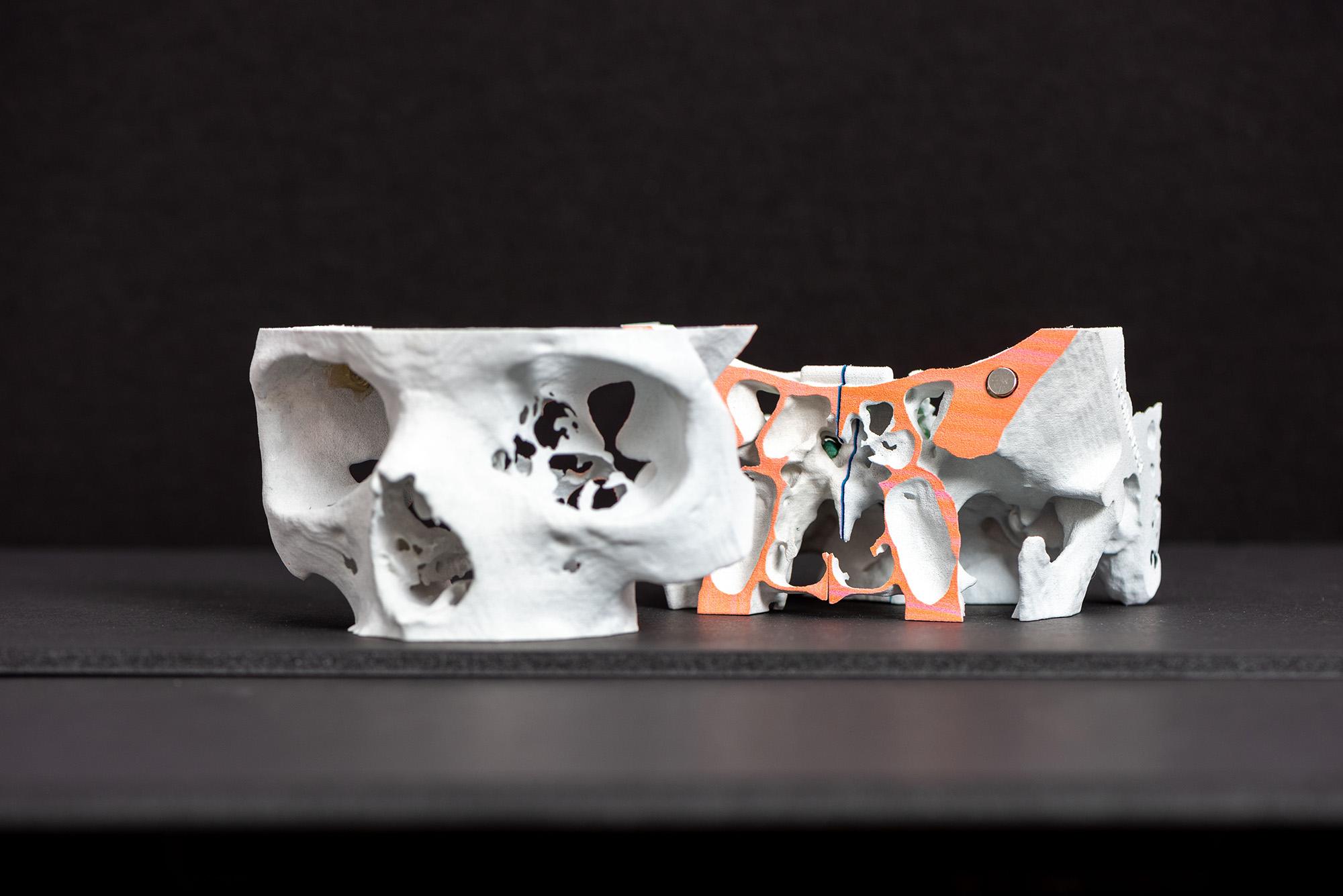
Innovation is the lifeblood of the medical field, which is constantly looking for new approaches, strategies, and tools to improve patient care, improve results, and streamline the delivery of healthcare. One of the more significant recent technology developments is 3D printing, or additive manufacturing, which has revolutionized the medical industry. It has paved the way for innovative approaches to intricate medical problems and stimulated research and development in a wide range of medical fields.
3D printing has historically been connected to sectors of the economy like manufacturing, fashion, and construction. However, the medical business, where these qualities can be most helpful, is beginning to understand and embrace its ability for customization, precision, and versatility. 3D printing has found use in the medical field for a variety of purposes, including the creation of accurate anatomical models for surgical planning, the fabrication of prosthetic limbs that are tailored, and even the bioprinting of tissue and organs.
Custom Prosthetics and Orthotics
The process of creating personalized orthotics and prosthetics is being revolutionized by advances in 3D printing technologies and advanced design tools. Conventional manufacturing methods, which were frequently expensive and time-consuming, depended on casting and molding techniques, which had inherent limits when it came to providing each patient with the best fit possible. However, 3D printing technology significantly reduces the requirement for labor-intensive apparatus and physical labor, resulting in rapid turnaround times and cost-effectiveness.
The distinct anatomy of every patient is scanned, and a digital model is created. Then, in collaboration with patients and bioengineers, clinicians create and tweak every aspect of the model to meet the unique requirements and aesthetic tastes of the user. When a 3D printer receives the generated digital file, it uses metals and resins to gradually transform into an actual thing. Unmatched customisation and accuracy are made possible by this technique, which enhances the fit and general health of the patient.
Furthermore, one crucial area where 3D printed orthotics and prosthetics excel over conventionally made alternatives is accessibility. 3D printed gadgets can reach underprivileged populations or developing nations where access to such medical care may be limited due to the decrease in cost and time. Thus, this technology has the potential to democratize access to these necessary medical gadgets.
Furthermore, these gadgets may be optimized and advanced far more quickly due to the iterative nature of digital design. Any improvements to the design or modifications to the patient's condition can be quickly and affordably included into the updated print, encouraging proactive adaptation to meet the changing demands of the individual.
A genuinely patient-centric approach is embodied by the interface of 3D printing technology with prostheses and orthotics, encouraging innovation in accessibility and care delivery. Benefits of 3D printed orthotics and prosthetics include rapid prototyping, personalization, and affordability, but their full potential has not yet been realized. The combination of these fields marks a paradigm change in healthcare, paving the way for more customized, reasonably priced, and easily accessible treatments.
Bioprinting and Tissue Engineering
In order to enhance regenerative therapies, the fields of biology, engineering, and medicine are merging through the use of tissue engineering and bioprinting. Generally speaking, bioprinting is a subfield of 3D printing that uses computer-controlled layer-by-layer deposition of biological materials (bioinks) to create intricate, three-dimensional (3D) biological structures.
Cells, extracellular matrix materials, and auxiliary elements that stimulate biological responses are the usual components of bioinks. These biologically-functional three-dimensional structures closely resemble the structure and functionality of natural tissues, making bioprinting an effective technique for tissue engineering.
Fundamentally, tissue engineering is concerned with creating biological replacements that mimic the properties of native human tissue. It seeks to use biological and material science technological breakthroughs to build artificial organ systems that can offer patients suffering from severe tissue damage or organ failure therapeutic alternatives. Significant advancements have been made in the sector in the areas of scaffold design, bioactive factor production, mechano-physiological cue application, and quality control for repeatability and scalability.
The accuracy of building the three-dimensional biological constructs is increased when bioprinting is combined with tissue engineering. Patient-specific organ transplants are a thing of the future because to this accuracy, which enables the development of geometry-specific tissues injected with patient-derived cells. The produced organoids can also be used as patient-specific models for toxicity and pharmacological efficacy testing, as well as for pathology research on diseases.
Technological developments in bioprinting have enhanced the fidelity and resolution of the printed constructions, including droplet deposition, micro extrusion, and laser-assisted bioprinting. The utilization of induced pluripotent stem cells (iPSCs) in conjunction with bioprinting has improved the ability to produce human-like tissues with functional vasculature and innervation, particularly in light of recent advancements in stem cell technology.
The maturing function of the bioprinted tissues is further ensured by the advancement of dynamic bioprinting techniques that mimic the inherent mechanical stimuli of the tissue environment.
Even with these developments, there are still a lot of obstacles in the way of completely utilizing bioprinting in tissue engineering. These include managing related ethical and regulatory challenges, enhancing vascularization, innervation, and immunological compatibility, and guaranteeing the long-term survival of bioprinted tissues and organs. The field will need to overcome these obstacles and advance toward therapeutic applications, which will require increased multidisciplinary research and collaborations.
Surgical Planning and Practice with AM
Surgery that is tailored to each patient is among the most revolutionary uses of AM in medicine. Surgeons can tailor surgical plans to each patient's specific anatomical shapes, sizes, and substrates using CT or MRI scans. 3D-printed models that are unique to each patient can be produced from these images once they have been converted into the DICOM (Digital Imaging and Communications in Medicine) standard. Bone, muscle, and blood arteries are examples of tissues with varying densities that can be represented by the color, material, and texturing. With the use of these models, surgeons may more fully understand and anticipate the best surgical intervention strategy, which reduces uncertainty and improvisation during the real process.
A level of precision and interaction made possible by additive manufacturing was previously unachievable. With the help of these accurate replicas, surgeons can now practice intricate surgical operations, improving patient safety by decreasing the possibility of unforeseen difficulties during the process. It offers a way to feel as well as see the goal structure, which is especially helpful for mentoring more junior surgeons and promoting patient-centered decision-making.
AM is essential in the development of patient-specific implants, including cranial plates and joint replacements, for specialties like as orthopedics, maxillofacial, and cardiothoracic surgery. Through precise sizing and form matching to the patient's anatomical region of interest, these implants enhance patient appropriateness, facilitate improved fit and alignment, and eventually yield improved long-term results.
Further promising for the future is AM's ability to print with biocompatible and resorbable materials like polycaprolactone (PCL), polylactic acid (PLA), or even a patient's own cells (bioprinting). A notable advancement in regenerative medicine is the use of biodegradable implants that promote the formation of native tissue or scaffolds that act as temporary frameworks for tissue engineering.
Last but not least, AM's progress in areas like bioprinting skin, cartilage, bone, and even organ structures has cleared the way to address issues with organ scarcity, lower the risk of graft-versus-host infections, and offer autologous alternatives that are better for patient recuperation. There is still a long way to go before a fully functional printed organ can be used, but there is hope that transplant rejection may become obsolete in the future.
Additive manufacturing in dentistry
AM has developed a novel method for producing precise, adaptable, and effective dental restorations. Additive manufacturing techniques are progressively replacing traditional processes, which are marked by a lot of effort and long fabrication times. Dental professionals are able to accurately diagnose dental diseases and create dental prostheses with precise dimensions and design thanks to the use of computer-aided design (CAD) and 3D imaging technologies. Additive manufacturing produces functioning dental constructions that are customized to each patient's specific oral anatomy by precisely adding portions layer by layer.
Dental operations like making crowns, bridges, braces, implants, retainers, and even whole sets of dentures have been greatly expedited with the use of additive manufacturing. Prostheses can now be produced internally by dental labs and clinics with short turnaround times and ideal fit, increasing patient comfort and satisfaction. By using this procedure, practitioners can also work with a broad range of biocompatible materials, such as metals, resins, and ceramics. Additive manufacturing also makes problem rectification easier since it allows for digital design adjustments that are precisely reflected in the finished product. These developments transform patient care procedures and hold out hope for a time when everyone will have access to individualized, excellent dental care.
Pharmaceuticals
The pharmaceutical sector has seen a considerable transformation due to the introduction of fresh ways for fabricating tailored medicines through 3D printing technology. This method makes it possible to provide medications tailored to each patient, accounting for factors including age, body weight, and illness severity. It assists in improving treatment efficacy and reducing side effects while overcoming the one-size-fits-all characteristic of traditional drug manufacture. For example, 3D-printed tablets may precisely control dosage and combine various medications into one pill, which reduces pill burden and improves patient compliance. In addition, the technique makes it easier to create intricate drug-release profiles, which enables the synthesis of medications with fast, slow, or delayed release characteristics in accordance with patient requirements.
One significant effect of 3D printing in the pharmaceutical industry is the fast evolving dynamics of the supply chain and drug manufacture. Decentralized drug manufacture is one possible future scenario. An age of on-demand pharmaceutical manufacturing may be ushered in by the possibility for 3D printing to enable the fabrication of medications at the point of administration or even at the patient's house. This could make the supply chain for pharmaceuticals less vulnerable to disruptions like those that occur during pandemics or natural disasters. Although this hypothetical future presents intriguing safety and regulatory issues, it has the potential to significantly improve healthcare accessibility and customization worldwide.
The Challenges in 3D printing Healthcare
The manufacturing of customized prostheses and patient-specific organ models for surgical planning are just two instances of how the healthcare industry is progressively incorporating 3D printing into many patient care domains. Still, there are many tough challenges ahead of us. Standardization and regulatory compliance rank among the main concerns since they are necessary to ensure patient safety. There are stringent regulations that apply to all 3D printed medical devices and may vary according on the location. Furthermore, there is currently a lack of a widely accepted set of standards for verifying the dependability, security, and performance of these printed goods. Consequently, navigating several oversight organizations and intricate regulatory frameworks could be challenging, which could obstruct the rapid innovation and broad adoption of 3D printing in the healthcare sector.
Furthermore, problems pertaining to the materials utilized in 3D printing pose a difficulty. Biocompatibility becomes crucial due to the variety of patient needs, particularly for implants and prosthesis that will come into close touch with the body. There is not a wide range of materials available, and creating new materials that are biocompatible and printable can be difficult and time-consuming. Furthermore, products produced by 3D printers need to be consistently precise and of high quality, especially when manufacturing delicate structures or items for use in medical settings. This means that the equipment must be extremely sophisticated and well-maintained, which presents serious financial and logistical difficulties.